In the realm of modern technology and everyday convenience, electrical circuits play an indispensable role in powering our world. These intricate networks of interconnected components facilitate the transmission and control of electrical energy, driving everything from the lighting in our homes to the operation of complex industrial machinery. Two fundamental concepts that lie at the heart of understanding the behavior of these circuits are power and energy.
Power, in the context of electrical circuits, is the rate at which energy is consumed or delivered. It quantifies the amount of work done per unit of time and provides a crucial insight into how efficiently a circuit operates. Understanding power is essential not only for designing and optimizing circuits but also for ensuring their safe and reliable operation.
Energy, on the other hand, is the capacity to do work. In electrical circuits, energy is stored and transferred through various components, such as resistors, capacitors, and inductors. A deep comprehension of energy dynamics is pivotal for determining the storage and conversion of electrical energy within a circuit, enabling us to harness and utilize electricity effectively.
This exploration of power and energy in electrical circuits will delve into the foundational principles that govern their behavior. From the mathematical relationships that define power and energy to practical applications and real-world examples, this journey will illuminate the intricate dance between these concepts and their impact on modern technology and daily life. Whether you’re a novice intrigued by the mysteries of circuits or an experienced engineer seeking to refine your understanding, a grasp of power and energy is essential for unraveling the mysteries of the electrical world.
Power in Electrical Circuits
Power is a fundamental concept in the world of electrical circuits, representing the rate at which energy is transferred, converted, or consumed within a circuit. It provides a key insight into how efficiently a circuit operates and plays a crucial role in the design, analysis, and optimization of electrical systems. Power is measured in watts (W), named after the famous Scottish inventor James Watt, and it has profound implications for various applications, from the everyday devices we use to the large-scale industrial systems that drive our economies.
Mathematical Definition: The power (P) in an electrical circuit can be mathematically defined as the product of voltage (V) and current (I):
P=V×I
Where:
- P is the power in watts (W).
- V is the voltage across the component in volts (V).
- I is the current flowing through the component in amperes (A).
This simple relationship highlights the direct correlation between voltage, current, and power. When voltage or current increases, power also increases proportionally.
Types of Power: In electrical circuits, power can be categorized into two main types: instantaneous power and average power.
- Instantaneous Power: This is the power at any given instant in time and is calculated by multiplying the instantaneous voltage and current. In alternating current (AC) circuits, both voltage and current vary sinusoidally over time, leading to variations in instantaneous power.
- Average Power: In cases where voltage and current vary over time, calculating the average power provides a more meaningful measure of energy consumption or delivery. For example, in an AC circuit, the average power is calculated over a complete cycle of the waveform. The average power is a crucial parameter for determining the energy efficiency of devices.
Significance of Power: Understanding power is essential for several reasons:
- Device Efficiency: Power indicates how efficiently an electrical device or component converts electrical energy into other forms of energy (such as light, heat, or mechanical motion). Inefficient devices may waste energy, leading to increased operational costs and environmental concerns.
- Circuit Analysis: Power analysis helps engineers and technicians assess the performance of electrical circuits. By analyzing power distribution and losses, they can identify areas for improvement or troubleshooting.
- Safety Considerations: High-power circuits can generate significant heat, posing safety risks. Understanding power helps ensure that circuits are designed with appropriate cooling mechanisms and insulation to prevent overheating and potential hazards.
- Energy Management: Power monitoring and management are crucial in applications like power distribution grids. Balancing power generation, transmission, and consumption ensures a stable and reliable supply of electricity.
- Renewable Energy: Power considerations are vital for renewable energy sources like solar panels and wind turbines. Monitoring power output helps assess their efficiency and integration into the overall power grid.
Power is a central concept in electrical circuits that quantifies the rate of energy transfer. Its impact extends across various domains, from domestic appliances to complex industrial systems, influencing the way we design, operate, and optimize electrical systems. A comprehensive understanding of power is essential for engineers, technicians, and anyone interested in the functioning of the technological world around us.
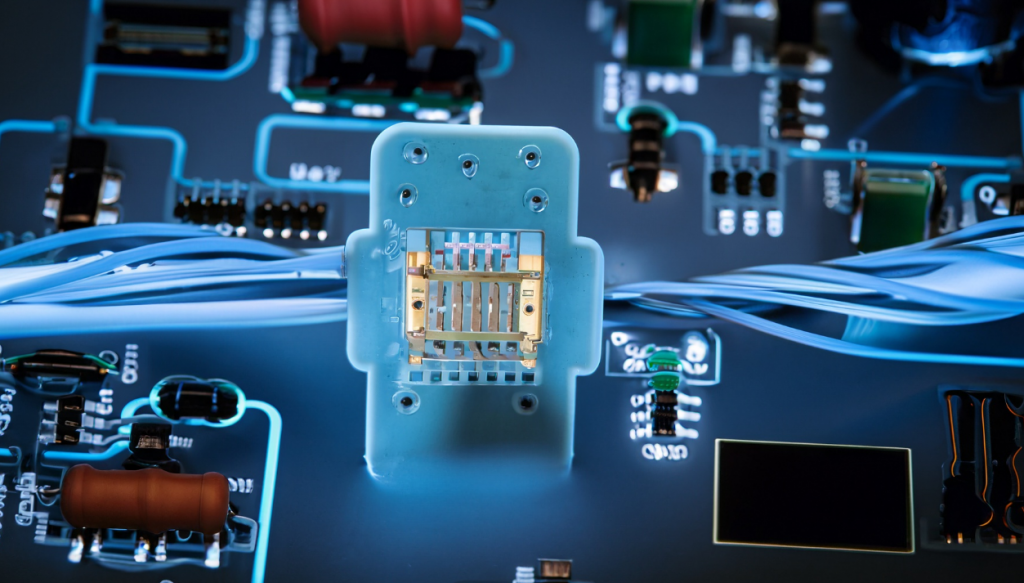
Energy in Electrical Circuits
Energy is a fundamental concept that underpins the operation of electrical circuits, serving as the driving force behind the functionality of various devices and systems. It encompasses the capacity to perform work and is transferred, stored, and transformed within electrical circuits, enabling the generation of light, heat, motion, and countless other forms of useful output. Understanding the principles of energy within circuits is essential for designing efficient systems, managing power resources, and ensuring the safe operation of electrical devices.
Types of Energy in Electrical Circuits:
- Electrical Energy: This is the energy associated with the movement of electric charges, typically in the form of electrons. Electrical energy is transferred through conductors, such as wires, and is the foundation of how electricity powers devices and systems.
- Potential Energy: In circuits, potential energy is stored when charges are separated by a voltage difference. This stored energy is released when charges move through a circuit, such as when a capacitor discharges or a battery powers a device.
- Magnetic Energy: Magnetic energy is associated with the magnetic fields generated by current-carrying conductors. This energy can be stored in inductors and released as current flows through them.
- Kinetic Energy: In some circuits, electrical energy is converted into kinetic energy, resulting in mechanical motion. For instance, electric motors transform electrical energy into rotational kinetic energy.
- Radiant Energy: Radiant energy is produced when electrical energy is converted into electromagnetic waves, as in the case of radio signals, light from bulbs, or microwave radiation.
Energy Conservation and Conversion:
The principle of energy conservation holds true in electrical circuits, just as it does in all physical systems. Energy is neither created nor destroyed but is merely transformed from one form to another. Understanding how energy is converted and distributed within circuits is vital for optimizing efficiency and minimizing losses.
Energy Storage Elements:
Certain circuit components have the ability to store energy temporarily, contributing to the dynamic behavior of circuits. Two common energy storage elements are capacitors and inductors.
- Capacitors: Capacitors store electrical energy in an electric field created by separated charges on two plates. When charged, they store potential energy, which can be released when the capacitor discharges.
- Inductors: Inductors store energy in a magnetic field generated by the flow of current. The energy is released as the magnetic field collapses when the current changes.
Calculating Energy:
The energy (E) stored in capacitors and inductors can be calculated using the following formulas:
- For a capacitor: E=1/2CV2, where C is the capacitance and V is the voltage across the capacitor.
- For an inductor:E=1/2LI2, where L is the inductance and I is the current through the inductor.
Applications and Importance:
Understanding energy in electrical circuits has numerous practical applications:
- Battery Life: Knowledge of energy storage and conversion helps predict the lifespan of batteries in various devices.
- Efficiency: By analyzing energy losses in circuits, engineers can design systems that operate with higher efficiency, reducing waste.
- Renewable Energy: Energy considerations are crucial for optimizing the output of renewable sources like solar panels and wind turbines.
- Safety: Understanding energy flow helps prevent hazards due to overloading or improper circuit designs.
In essence, energy is the lifeblood of electrical circuits, enabling them to perform a wide array of functions that power our modern world. Its proper management and utilization are key to creating sustainable and efficient electrical systems.
Active and Passive Components
Electrical circuits are composed of a variety of components that work together to create functional systems. These components can be broadly classified into two main categories: active components and passive components. Each category plays a distinct role in circuit behavior, contributing to the transmission, control, and manipulation of electrical signals and energy.
Active Components:
Active components are elements within a circuit that are capable of amplifying, switching, or controlling the flow of electrical current. These components require an external source of power to operate and are capable of adding energy to a circuit. Active components are essential for signal processing, amplification, and creating complex functionalities in electronic systems.
Some common examples of active components include:
- Transistors: Transistors are fundamental active components used for amplification and switching. They can be configured to control current flow between two terminals based on a small input signal.
- Operational Amplifiers (Op-Amps): Op-amps are versatile integrated circuits that are widely used for amplification, filtering, and signal conditioning. They have a high input impedance and can provide high gain.
- Integrated Circuits (ICs): ICs consist of multiple interconnected active and passive components on a single chip. They can perform a wide range of functions, from logic operations to signal processing.
- Diodes (in some applications): While diodes are primarily passive components, some diodes, like light-emitting diodes (LEDs) and laser diodes, exhibit active behavior by emitting light when forward-biased.
Passive Components:
Passive components, unlike active components, do not require an external power source and do not amplify or control signals. Instead, they modify the characteristics of electrical signals passing through them, such as resistance, capacitance, and inductance. Passive components are crucial for shaping signals, filtering unwanted frequencies, and providing impedance matching.
Some common examples of passive components include:
- Resistors: Resistors limit the flow of current in a circuit, converting electrical energy into heat. They are used for voltage division, current limiting, and signal attenuation.
- Capacitors: Capacitors store and release electrical energy in the form of an electric field. They are used for energy storage, coupling signals between different parts of a circuit, and filtering out low-frequency components.
- Inductors: Inductors store energy in a magnetic field when current flows through them. They resist changes in current and are used in applications like filtering, energy storage, and creating time delays.
- Transformers: Transformers are devices that use the principle of electromagnetic induction to transfer electrical energy between different circuits. They are often used to step up or step down voltage levels in power distribution.
- Passive Filters: Passive filters, made up of combinations of resistors, capacitors, and inductors, are used to selectively pass or block certain frequencies in a signal.
Active components introduce energy and control into electrical circuits, while passive components modify the characteristics of signals without introducing additional energy. Both active and passive components are essential building blocks in the design of electronic systems, allowing engineers to create complex circuits with specific functions and behaviors.
Power Losses and Efficiency
Efficiency and power losses are crucial considerations in the design and operation of electrical circuits and systems. These concepts provide insights into how effectively electrical energy is converted, transmitted, and utilized within a circuit, influencing the performance, sustainability, and cost-effectiveness of various applications.
Power Losses:
Power losses occur due to various factors within an electrical circuit, leading to the dissipation of energy in the form of heat. These losses can occur in both active and passive components and are categorized into several types:
- Conduction Losses: These losses occur due to the resistance of conductive materials, such as wires, connectors, and traces on circuit boards. As current flows through these materials, some of the energy is converted into heat.
- Switching Losses: Active components like transistors and diodes have finite switching times. During transitions between on and off states, there can be brief moments when both voltage and current are non-zero, resulting in switching losses.
- Dielectric Losses: Capacitors, which store energy in an electric field, can experience energy losses due to the resistance of the dielectric material between their plates.
- Magnetic Core Losses: Transformers and inductors with magnetic cores can experience losses due to hysteresis (magnetic domains resisting change) and eddy currents (localized circulating currents).
- Radiation Losses: High-frequency circuits can radiate electromagnetic energy, leading to energy losses in the form of radio waves. This is especially relevant in applications like wireless communication.
Efficiency:
Efficiency (η) is a measure of how well a circuit or system converts input energy into useful output energy while minimizing losses. It is expressed as a percentage and is calculated using the formula:
η=Useful Output PowerInput Power×100%η=Input PowerUseful/Output Power×100%
A high-efficiency system minimizes wasteful energy losses, resulting in better performance and reduced operating costs. However, achieving high efficiency might involve trade-offs in terms of complexity, size, and cost of components.
Factors Affecting Efficiency:
- Component Selection: Choosing components with lower resistance, faster switching times, and higher quality materials can reduce losses.
- Design: Proper circuit layout, heat sinking, and shielding can minimize energy losses due to conduction and radiation.
- Operating Conditions: Running components within their specified ratings and avoiding excessive current or voltage levels can reduce losses.
- Cooling: Efficient cooling mechanisms prevent components from overheating, maintaining their optimal performance and reducing losses.
Applications:
Efficiency and power loss considerations are critical in various applications:
- Energy Conversion Systems: In power supplies, inverters, and converters, high efficiency reduces energy waste and heat generation.
- Renewable Energy: Efficient energy conversion is essential in solar panels, wind turbines, and energy storage systems to harness maximum power from renewable sources.
- Transportation: Efficient motor drives and power management systems in electric vehicles increase range and reduce energy consumption.
- Industrial Applications: Industrial machines and processes benefit from efficient motor control and power distribution systems.
- Consumer Electronics: Efficient power management extends battery life in portable devices.
Power losses and efficiency are critical factors in the design, operation, and sustainability of electrical circuits and systems. Minimizing losses through proper component selection, design, and operating conditions can lead to more reliable, cost-effective, and environmentally-friendly solutions across a wide range of applications.
Three-Phase Power Systems
Three-phase power systems are a fundamental and widely used method of distributing electrical power in industrial, commercial, and residential applications. Unlike single-phase systems, which involve a single alternating current (AC) waveform, three-phase systems utilize three separate AC waveforms that are synchronized in frequency and amplitude. This configuration offers several advantages, including higher power transmission efficiency, balanced loads, and reduced voltage fluctuations. Three-phase systems are commonly found in power generation plants, transmission lines, motors, and various industrial machines.
Key Concepts:
- Phases and Phasors: A three-phase system consists of three phases: Phase A, Phase B, and Phase C. Each phase has its own AC waveform, typically sinusoidal, with a 120-degree phase difference between adjacent phases. Phasors are used to represent the magnitudes and relative angles of the three phases.
- Line and Phase Voltages: In a three-phase system, there are two voltage measurements – line voltage (V_L) and phase voltage (V_P). The line voltage is the voltage between any two phases, while the phase voltage is the voltage between a phase and the system’s neutral point.
- Balanced Loads: Ideally, three-phase loads are balanced, meaning they draw equal currents from each phase. This balanced behavior minimizes neutral current and optimizes system efficiency.
- Delta and Wye (Star) Configurations: Loads can be connected in either a delta (Δ) or a wye (star) (Y) configuration. In a delta configuration, each load is connected between two phases. In a wye configuration, the loads are connected between phases and a common neutral point.
Advantages:
- Higher Power Capacity: Three-phase systems deliver more power compared to single-phase systems of the same voltage level. This is essential for powering heavy machinery and large industrial systems.
- Efficiency: Three-phase systems have a more uniform distribution of power, leading to lower line losses and better energy efficiency.
- Balanced Loads: Balanced loads reduce the neutral current, which is particularly important in wye configurations where the neutral conductor can be smaller in size.
- Constant Power Transfer: In three-phase systems, the power transferred remains nearly constant over time, leading to stable performance in machines and equipment.
Applications:
Three-phase power systems find extensive use in various applications:
- Power Generation: Many power plants generate electricity in three-phase systems. This configuration facilitates efficient power transmission and distribution.
- Power Transmission: Electricity is transmitted over long distances using high-voltage three-phase transmission lines, which reduces power losses during transmission.
- Motors: Three-phase induction motors are common in industrial settings due to their reliability and efficiency.
- Industrial Machines: Machines like pumps, compressors, and fans benefit from the constant power delivery of three-phase systems.
- Commercial Buildings: Large commercial buildings use three-phase systems for lighting, heating, ventilation, and air conditioning (HVAC) systems.
- Renewable Energy: Wind turbines and solar inverters often use three-phase systems to convert and distribute generated energy.
In conclusion, three-phase power systems offer numerous advantages, including higher power capacity, better efficiency, and balanced loads. Their widespread use in industrial and commercial applications demonstrates their importance in modern electrical engineering, ensuring reliable and efficient power distribution for a multitude of purposes.
Conclusion
In the intricate world of electrical circuits, the concepts of power and energy stand as the pillars that support our modern technological landscape. Power, the rate at which energy is consumed or delivered, provides a lens through which we understand the efficiency and functionality of circuits. It guides engineers in designing systems that optimize energy usage and minimize wasteful losses. Energy, the capacity to do work, flows through circuits, transforming into various forms that power our lives, from the lighting that brightens our surroundings to the devices that keep us connected.
The synergy between power and energy is crucial for comprehending the behavior of electrical circuits. The relationship between voltage, current, and power lays the groundwork for calculations and optimizations, ensuring circuits operate within safe and efficient limits. Understanding the dynamics of energy storage and conversion, whether through capacitors, inductors, or other components, empowers us to harness electricity’s potential for various applications.
As technology advances, the demand for energy-efficient solutions becomes more pressing. The principles of power and energy play a pivotal role in addressing this challenge. By striving for high efficiency, minimizing losses, and making informed choices about component selection and system design, we can create circuits that perform optimally while reducing environmental impact.
Whether you’re an engineer designing intricate electronic systems, a student exploring the foundations of electrical circuits, or a consumer benefiting from the marvels of modern technology, a grasp of power and energy in electrical circuits is paramount. These concepts not only illuminate the underlying mechanisms of our interconnected world but also offer the tools to create a future where electricity is harnessed more intelligently, sustainably, and effectively.