In the intricate realm of electronics and signal processing, filters and amplifiers stand as foundational pillars, shaping the way information flows and is transformed within circuits. Filters, akin to gatekeepers, allow specific frequencies to pass through while attenuating others, enabling us to sieve and manipulate signals according to our needs. On the other hand, amplifiers act as signal boosters, elevating the strength of weak input signals to desired levels, propelling the efficacy of electronic systems. These two components, often working in tandem, find application in a plethora of domains, ranging from audio and telecommunications to medical instrumentation and beyond. Exploring the principles, types, and applications of filters and amplifiers unravels a captivating journey into the core of modern electronics, where control and enhancement of signals reign supreme.
Types of Filters
Filters are essential components in electronics and signal processing that allow certain frequencies to pass through while attenuating or blocking others. They play a crucial role in shaping the characteristics of signals by selectively modifying their frequency content. Filters are broadly categorized into several types based on their frequency response and design characteristics. Here are some of the main types of filters:
- Low-Pass Filters (LPF): Low-pass filters allow frequencies below a certain cutoff frequency to pass through while attenuating higher frequencies. They are commonly used to eliminate high-frequency noise from signals and to extract the lower-frequency components. Low-pass filters find applications in audio systems, anti-aliasing in digital signal processing, and communication systems.
- High-Pass Filters (HPF): High-pass filters, in contrast, allow frequencies above a cutoff frequency to pass through while attenuating lower frequencies. These filters are useful for removing low-frequency noise or DC offset from signals, and they are employed in audio equalization, AC coupling, and some types of image processing.
- Band-Pass Filters (BPF): Band-pass filters permit a specific range of frequencies, known as the passband, to pass through while attenuating frequencies outside this range. They are effective for extracting specific frequency components from a signal and are commonly used in radio communication, audio equalization, and medical signal processing.
- Band-Stop Filters (BSF) or Notch Filters: Band-stop filters, also known as notch filters, attenuate a certain range of frequencies, creating a “notch” in the frequency response. These filters are employed to suppress specific interference frequencies or to remove unwanted noise spikes from signals. They are used in applications like power line noise reduction and audio feedback elimination.
- All-Pass Filters: All-pass filters are designed to allow all frequencies to pass through, but they alter the phase relationships between different frequency components. They find use in applications such as phase correction and audio equalization, where altering the phase response is desired without affecting the magnitude response.
- Butterworth Filters: Butterworth filters are known for providing a maximally flat frequency response in the passband while gradually rolling off towards the stopband. They are commonly used in applications where a smooth transition between passband and stopband is important.
- Chebyshev Filters: Chebyshev filters offer steeper roll-off characteristics compared to Butterworth filters, but with some ripples in either the passband or stopband. They are useful when a sharper cutoff is required and some variation in the frequency response can be tolerated.
- Elliptic Filters (Cauer Filters): Elliptic filters provide the steepest roll-off of all filter types, but they exhibit ripples in both the passband and stopband. These filters are used in applications where stringent control over the frequency response is crucial.
The choice of filter type depends on the specific requirements of the application, including the desired frequency response, roll-off characteristics, and tolerance for ripples. Filters play a fundamental role in signal processing, telecommunications, audio systems, and a wide range of other electronic applications, enabling the manipulation of signals to meet diverse needs.
Applications of Filters
Filters are versatile components used in a wide array of applications across various fields, ranging from telecommunications and audio to image processing and scientific research. Their ability to selectively modify the frequency content of signals makes them indispensable for shaping and enhancing the quality of signals in numerous ways. Here are some prominent applications of filters:
- Audio and Music: Filters are extensively used in audio systems for equalization, noise reduction, and audio effects. Low-pass and high-pass filters help create balanced audio output by removing unwanted frequency extremes. Band-pass filters are employed to separate different instrument frequencies, while notch filters can eliminate specific hums or unwanted background noises.
- Wireless Communication: Filters are crucial in wireless communication systems to isolate different frequency bands and prevent interference. They are used in cellular networks, Wi-Fi routers, and radio transceivers to ensure that signals of interest are transmitted and received without distortion.
- Image and Video Processing: Filters find applications in image and video processing to enhance or manipulate visual content. In image editing, filters can blur, sharpen, or edge-detect images, while in video compression, filters help reduce data by removing redundant information while preserving important details.
- Biomedical and Medical Instruments: Filters play a vital role in medical devices for monitoring and analyzing physiological signals. Electrocardiograms (ECGs), electroencephalograms (EEGs), and other bioelectric signals often require filtering to eliminate noise and isolate relevant frequency components for accurate diagnosis.
- Radar and Sonar Systems: In radar and sonar systems, filters help distinguish between different types of targets by separating echoes from background noise. These filters ensure that only echoes corresponding to specific ranges of frequencies are processed, improving target detection and identification.
- Acoustic Engineering: In architectural acoustics and sound reinforcement systems, filters are used to control the frequency response of audio signals in order to achieve desired sound characteristics within spaces like concert halls, auditoriums, and theaters.
- Environmental Monitoring: Filters are utilized in environmental monitoring systems to isolate specific pollutants or gases from complex mixtures of gases and particles. This enables accurate detection and measurement of contaminants in the air or water.
- Analog and Digital Signal Processing: Filters are a cornerstone of signal processing, both in analog and digital domains. In analog applications, filters can clean up audio signals before they are digitized. In the digital realm, they are used for various tasks such as noise reduction, data smoothing, and data compression.
- Power Electronics: Filters are employed in power electronics to reduce harmonics and noise in electrical systems, ensuring the stability and efficiency of power distribution networks. They are often used in conjunction with converters and inverters to improve power quality.
- Astronomy and Radio Telescopes: In astronomy, filters are utilized to isolate specific wavelengths of light from celestial objects, allowing scientists to study individual spectral lines and gather information about the composition and characteristics of astronomical phenomena.
These applications merely scratch the surface of the vast utility of filters in modern technology. Filters are essential tools that enable us to tailor and optimize signals to meet the requirements of various applications, making them indispensable in countless aspects of our interconnected world.
Types of Amplifiers
Amplifiers are electronic devices that increase the amplitude or strength of a signal, making them crucial components in various applications, including audio systems, communication systems, instrumentation, and more. Amplifiers come in different types, each designed to suit specific requirements and applications. Here are some of the main types of amplifiers:
- Voltage Amplifiers: Voltage amplifiers, also known as voltage gain amplifiers, increase the voltage level of an input signal while maintaining its shape. They are commonly used in audio systems, where they boost the weak output of audio sources like microphones, guitars, and CD players to a level suitable for driving speakers.
- Current Amplifiers: Current amplifiers increase the current level of an input signal while keeping its voltage relatively constant. These amplifiers find applications in areas such as sensor signal conditioning and certain industrial automation processes.
- Power Amplifiers: Power amplifiers are designed to provide a significant increase in both voltage and current levels, enabling them to drive high-power output devices such as loudspeakers, motors, and actuators. They are crucial components in audio amplification, where they deliver the necessary power to produce sound at audible levels.
- Operational Amplifiers (Op-Amps): Operational amplifiers are versatile integrated circuits used in a wide range of applications, from basic signal conditioning to complex mathematical operations. They are often configured as voltage amplifiers, summing amplifiers, integrators, differentiators, and more.
- Differential Amplifiers: Differential amplifiers amplify the difference between two input signals while rejecting common-mode signals (signals present in both inputs). They are used in applications where noise rejection and signal differential analysis are crucial, such as in instrumentation and communication systems.
- Class A Amplifiers: Class A amplifiers operate in such a way that the output transistors are always conducting, even when there is no input signal. While they offer high linearity and low distortion, they are relatively inefficient due to continuous power consumption.
- Class B Amplifiers: Class B amplifiers use two transistors that conduct alternately during different halves of the input signal cycle. They are more efficient than Class A amplifiers but suffer from crossover distortion near zero input levels.
- Class AB Amplifiers: Class AB amplifiers combine characteristics of Class A and Class B amplifiers to achieve a balance between efficiency and linearity. They are commonly used in audio amplification to reduce crossover distortion while still maintaining good efficiency.
- Class D Amplifiers: Class D amplifiers, also known as switching amplifiers, use pulse-width modulation (PWM) to deliver high-efficiency amplification by rapidly switching the output devices between on and off states. They are commonly used in audio applications and are especially efficient for driving speakers.
- Class G and Class H Amplifiers: These are variations of Class AB amplifiers that use multiple power supply levels to increase efficiency. Class G amplifiers switch between different supply voltages based on the input signal level, while Class H amplifiers dynamically adjust the supply voltage in real time.
- Instrumentation Amplifiers: Instrumentation amplifiers are specialized amplifiers designed to provide high input impedance, high common-mode rejection, and high accuracy in amplifying small differential signals. They are used in applications where accurate signal acquisition and noise rejection are critical, such as medical instrumentation and sensor interfaces.
- Isolation Amplifiers: Isolation amplifiers provide electrical isolation between input and output while amplifying the signal. They are used in applications where electrical isolation is needed to prevent ground loops and noise interference, such as in industrial control systems and medical devices.
Each type of amplifier serves specific purposes and offers unique characteristics, making it important to choose the right amplifier type based on the application’s requirements for gain, efficiency, linearity, and other performance factors.
Amplifier Parameters and Characteristics
Amplifiers are essential components in electronics, serving to increase the strength of signals. To understand their performance and suitability for different applications, it’s important to consider various amplifier parameters and characteristics. These parameters provide insights into an amplifier’s behavior, efficiency, linearity, and other critical aspects. Here are some key amplifier parameters and characteristics:
- Gain (Voltage Gain, Current Gain, Power Gain): Gain represents the ratio of output amplitude to input amplitude. For voltage amplifiers, it’s the voltage gain (Av), for current amplifiers, it’s the current gain (Ai), and for power amplifiers, it’s the power gain (Ap). Gain determines how much an amplifier magnifies the input signal.
- Bandwidth: The bandwidth of an amplifier is the range of frequencies over which it can amplify signals effectively without significant attenuation. It’s often specified as the frequency range between the points where the gain drops by a certain percentage from its maximum value (e.g., -3 dB points).
- Frequency Response: The frequency response is a graphical representation of an amplifier’s gain as a function of frequency. It shows how the amplifier’s gain changes across different frequency ranges. A flat frequency response indicates that the amplifier has consistent gain across its specified frequency range.
- Input and Output Impedance: Input impedance (Zin) is the impedance presented by the amplifier’s input terminals to the signal source, while output impedance (Zout) is the impedance seen by the load connected to the amplifier’s output. Matching impedance between components is important for efficient signal transfer and power delivery.
- Linearity and Distortion: Linearity refers to an amplifier’s ability to accurately reproduce the output signal with minimal distortion. Distortion can introduce harmonics or alter the shape of the output waveform compared to the input waveform. Low distortion is essential for faithful signal reproduction, especially in audio applications.
- Sensitivity: Sensitivity measures the change in output signal for a given change in input signal level. It helps determine an amplifier’s responsiveness to small input variations. Higher sensitivity values indicate greater responsiveness.
- Noise and Signal-to-Noise Ratio (SNR): Noise in an amplifier introduces unwanted random fluctuations in the output signal. A high SNR indicates that the desired signal is significantly larger than the noise, which is important for maintaining signal quality in sensitive applications.
- Power Efficiency: Power efficiency quantifies how effectively an amplifier converts input power into output power. It’s a crucial parameter for power amplifiers, where minimizing energy waste is important.
- Common-Mode Rejection Ratio (CMRR): CMRR measures an amplifier’s ability to reject common-mode signals (signals present in both input terminals) while amplifying the differential signal (difference between the two inputs). High CMRR is important in differential amplifiers and instrumentation amplifiers to eliminate common-mode noise.
- Slew Rate: Slew rate represents the maximum rate at which an amplifier’s output voltage can change in response to a step input signal. It determines an amplifier’s ability to accurately reproduce fast-changing signals without distortion.
- Output Offset Voltage: Output offset voltage is the DC voltage that appears at the output of an amplifier when there is no input signal. Minimizing offset voltage is important to prevent unwanted DC shifts in the amplified signal.
- Temperature Stability: Amplifiers’ characteristics can change with temperature variations. Good temperature stability ensures that an amplifier’s performance remains consistent across different operating conditions.
Understanding these parameters and characteristics is essential for selecting the right amplifier for a given application and ensuring optimal performance. The choice of amplifier type and its parameters depends on the specific requirements of the application, such as signal level, frequency range, distortion tolerance, and power efficiency.
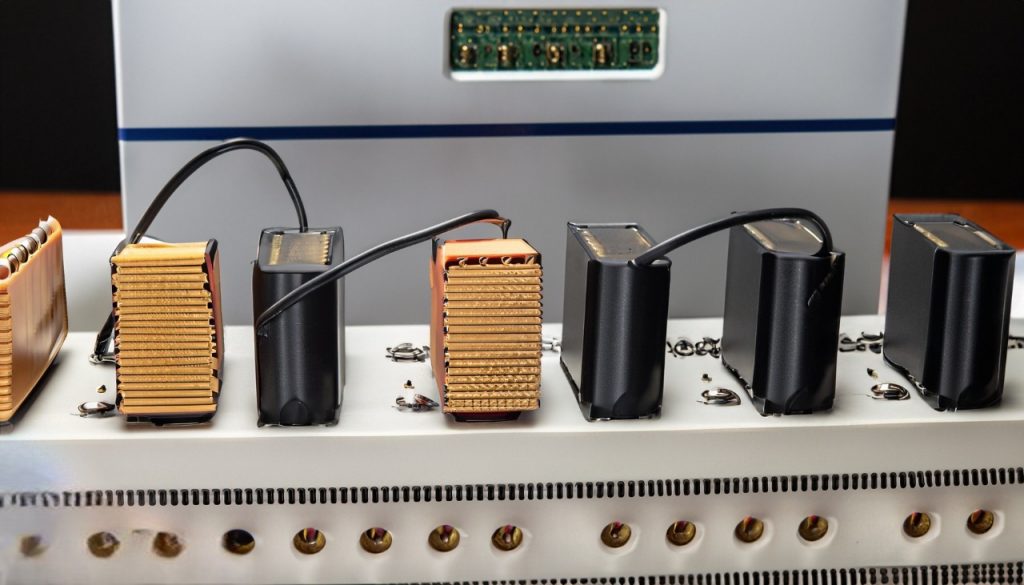
Amplifier Circuit Configurations
Amplifier circuit configurations define how amplifier components like transistors and operational amplifiers (op-amps) are interconnected to achieve specific amplification characteristics. Different configurations are tailored to suit various applications, providing advantages in terms of gain, input/output impedance, linearity, and other performance factors. Here are some common amplifier circuit configurations:
- Common Emitter Amplifier: This configuration is commonly used for bipolar junction transistor (BJT) amplifiers. The common emitter amplifier provides high voltage gain and moderate input impedance. It’s widely used in audio amplifier stages due to its good balance between gain and distortion.
- Common Collector Amplifier (Emitter Follower): Also known as the emitter follower, this configuration offers unity voltage gain but provides high current gain. It has a low input impedance and high output impedance, making it useful as a buffer between stages with different impedance levels.
- Common Base Amplifier: The common base amplifier provides low input impedance and voltage gain less than unity. It’s often used in applications requiring low noise and high-frequency response, such as RF amplifiers.
- Differential Amplifier: Differential amplifiers amplify the difference between two input signals while rejecting common-mode signals. They are used for noise rejection and precise signal amplification in instrumentation and communication systems.
- Operational Amplifier (Op-Amp): Op-amps are versatile integrated circuits that can be configured in various ways, including inverting amplifier, non-inverting amplifier, integrator, differentiator, and more. Op-amp configurations are used for a wide range of signal processing tasks due to their high gain, high input impedance, and low output impedance.
- Darlington Pair Amplifier: The Darlington pair involves cascading two transistors to achieve high current gain. This configuration is used when extremely high current gain is required, such as in driving high-power loads.
- Cascode Amplifier: The cascode configuration combines a common-emitter stage with a common-base stage. It provides high input impedance, high voltage gain, and improved bandwidth compared to a single-transistor amplifier.
- Push-Pull Amplifier: Push-pull amplifiers utilize complementary transistors (NPN and PNP) to amplify both halves of an AC waveform. They provide high efficiency and are commonly used in audio power amplifiers to deliver power to speakers.
- Class-D Amplifier: A class-D amplifier uses pulse-width modulation to achieve high-efficiency amplification. It switches rapidly between two discrete output levels, resulting in less power dissipation and higher efficiency.
- Transformer-Coupled Amplifier: Transformer-coupled amplifiers use transformers to couple the input and output signals. They are used in high-voltage applications and when galvanic isolation is needed.
- Instrumentation Amplifier: This specialized amplifier configuration provides high input impedance, low output impedance, and excellent common-mode rejection. It’s used in applications requiring precise amplification of small differential signals, such as medical instrumentation and sensors.
- Feedback Amplifier: Feedback configurations, such as voltage feedback and current feedback, use feedback networks to adjust the amplifier’s characteristics. Feedback amplifiers offer improved stability, linearity, and reduced distortion.
The choice of amplifier configuration depends on the specific requirements of the application, including desired gain, input/output impedance, bandwidth, distortion tolerance, and efficiency. Understanding these configurations allows engineers to design amplifiers tailored to specific tasks and optimize their performance for various applications.
Applications of Amplifiers
Amplifiers play a crucial role in a wide range of applications across various industries, enabling the enhancement and manipulation of signals to meet specific needs. Their ability to amplify weak signals while maintaining their integrity is instrumental in shaping modern technology. Here are some notable applications of amplifiers:
- Audio Systems: Amplifiers are extensively used in audio systems, from small headphones to high-power sound reinforcement systems. They amplify signals from sources like microphones, musical instruments, and media players to produce clear and loud sound through speakers.
- Telecommunications: Amplifiers are essential in telecommunications networks to boost signals as they travel long distances through fiber-optic cables, coaxial cables, and wireless channels. They’re used in cell towers, satellite communication, and undersea cables to maintain signal strength.
- Wireless Communication: In wireless systems, amplifiers are crucial for transmitting and receiving signals. They’re used in RF (Radio Frequency) amplifiers to increase the power of signals before they are transmitted, as well as in low-noise amplifiers to boost weak incoming signals.
- Medical Instruments: Amplifiers are used in medical devices for amplifying bioelectric signals such as electrocardiograms (ECGs), electroencephalograms (EEGs), and electromyograms (EMGs). These amplifiers help healthcare professionals diagnose medical conditions accurately.
- Instrumentation and Measurement: In scientific research and industrial applications, amplifiers are used to amplify signals from sensors and transducers, ensuring accurate data acquisition. Instrumentation amplifiers provide high input impedance and accurate amplification for precise measurements.
- Audio Equipment Manufacturing: The manufacturing of audio equipment, such as headphones, speakers, and audio interfaces, heavily relies on amplifiers to drive transducers and produce quality sound reproduction.
- Power Amplifiers in Electric Vehicles: Amplifiers are used in electric vehicles (EVs) to amplify the low-power audio signals for speakers. Additionally, they’re used in motor drive circuits to control the electric motor, providing efficient propulsion.
- Guitar Amplifiers: Musicians use guitar amplifiers to amplify the sound produced by electric guitars. These amplifiers enhance the tonal characteristics of the guitar and allow musicians to shape their sound through various controls and effects.
- Aerospace and Defense: Amplifiers are used in radar systems, communication equipment, and electronic warfare systems. They enable the transmission of signals over long distances and help in detecting and processing weak signals.
- Amplified Musical Performances: Amplifiers are crucial in live music performances, allowing musicians to project their instruments and vocals to a larger audience. Amplifiers for guitars, basses, keyboards, and microphones are standard equipment in concert setups.
- Industrial Automation: In industrial settings, amplifiers are used in motor control applications to drive motors and actuators efficiently, enabling precise control of machinery and processes.
- Consumer Electronics: Amplifiers are found in a variety of consumer electronics, including TVs, home theater systems, gaming consoles, and portable audio devices, enhancing the audio-visual experience.
- Sonar and Radar Systems: Amplifiers are critical in sonar and radar systems for amplifying the return signals from objects and targets, aiding in target detection and localization.
These applications merely scratch the surface of the extensive role amplifiers play in modern technology and everyday life. Their ability to amplify and shape signals has led to advancements in diverse fields, enabling improved communication, precision measurement, entertainment, and more.
Conclusion
In the intricate landscape of electronics and signal processing, filters and amplifiers stand as fundamental tools that shape the way we interact with and manipulate signals. Filters, akin to skilled curators, refine the content of signals, allowing us to extract specific frequencies of interest while attenuating unwanted noise. Meanwhile, amplifiers, like master artisans, elevate the power and amplitude of signals, unlocking their potential to drive everything from audio systems to communication networks.
The diverse types of filters—low-pass, high-pass, band-pass, and band-stop—serve as gatekeepers, ensuring that signals conform to desired frequency ranges. Their applications span from audio enhancement and noise reduction to precise medical diagnostics and efficient signal transmission. Filters offer the means to sculpt signals to meet the unique demands of each context, from audio reproduction to scientific research.
Likewise, amplifier configurations—common emitter, differential, operational, and many more—act as sculptors, molding signals into forms suited for different tasks. Amplifiers find their roles in myriad domains, from audio amplification and telecommunications to space exploration and medical advancements. By boosting signal strengths and enabling accurate replication, amplifiers underpin the functionality of various electronic systems.
Together, filters and amplifiers create a symphony of technological innovation. They work in harmony to refine, elevate, and propagate signals through a vast array of applications, transcending the limits of sound, communication, and information processing. As the world of electronics continues to evolve, the mastery of filters and amplifiers remains an essential art that shapes the way we perceive, communicate, and explore the realms of both the audible and the invisible.