In the realm of modern electronics, transistors stand as fundamental building blocks that have revolutionized the way electronic devices operate. These small semiconductor devices serve as the cornerstone of digital circuits, enabling amplification, switching, and signal processing with unparalleled precision and efficiency. Since their inception, transistors have undergone remarkable advancements, leading to the development of various types, each tailored to specific applications. This introduction delves into the world of transistors, exploring their significance, basic functionality, and the diverse types that have shaped the landscape of contemporary technology.
Transistors, often referred to as the “brains” behind modern electronics, are semiconductor devices that control the flow of electric current between two terminals. They play a pivotal role in amplifying weak signals, acting as switches to control the flow of current, and forming the basis for integrated circuits that power everything from smartphones to supercomputers. Their invention in the mid-20th century marked a turning point in electronics, supplanting the bulkier and less efficient vacuum tubes that dominated earlier electronic designs.
The basic functionality of a transistor relies on its ability to regulate the movement of electrons across its terminals, primarily achieved through the manipulation of charge carriers within a semiconductor material. By using a small input signal to control a much larger output signal, transistors provide the means to amplify and control electronic signals, making them indispensable in various applications ranging from radios and televisions to medical devices and space exploration.
Over time, transistors have undergone significant evolution, leading to the development of multiple types, each catering to specific requirements. Bipolar Junction Transistors (BJTs), for instance, offer high current gain and are adept at amplification tasks, while Field-Effect Transistors (FETs) excel in high-input impedance scenarios and serve as efficient switches. Metal-Oxide-Semiconductor FETs (MOSFETs) further subdivided into N-channel and P-channel types, have emerged as the backbone of modern integrated circuits due to their low power consumption and robust performance.
Other variations, such as Junction Field-Effect Transistors (JFETs), Insulated-Gate Bipolar Transistors (IGBTs), and many more, continue to expand the possibilities of electronics in various industries. From consumer electronics to telecommunications, automotive systems to renewable energy technologies, transistors in their various forms continue to shape the way we interact with and harness the power of electricity.
Transistors represent a cornerstone of modern electronics, enabling a myriad of technological innovations that define our daily lives. Their ability to amplify, switch, and process electronic signals efficiently has paved the way for the creation of smaller, more powerful, and energy-efficient devices. As we delve deeper into the world of transistors and explore their diverse types, we uncover the intricate web of technology that underpins our interconnected world.
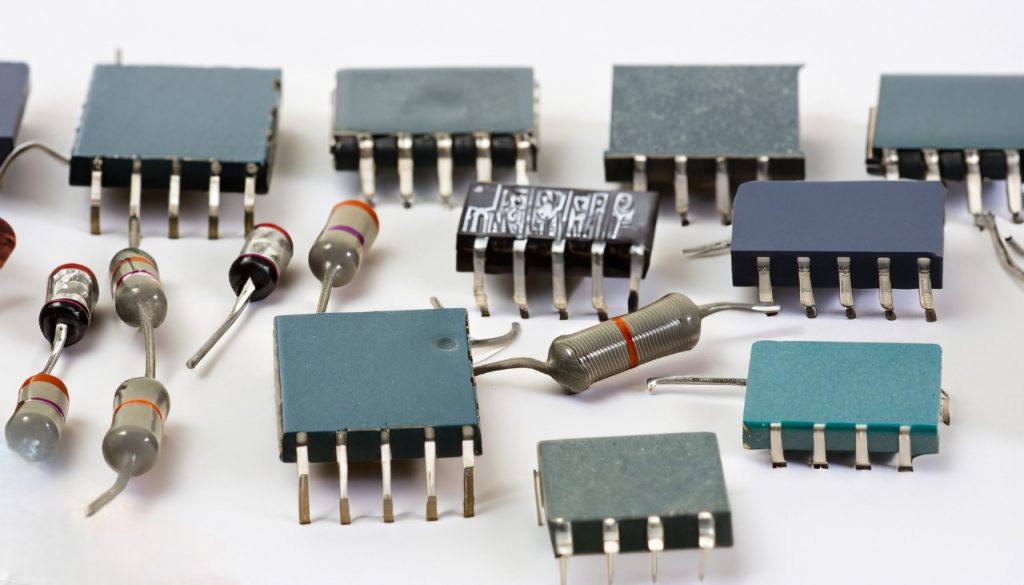
Working Principle of Transistors
The working principle of transistors is rooted in the behavior of semiconductors and the manipulation of their charge carriers. Transistors are devices that regulate the flow of electric current between two terminals, known as the emitter and the collector, by controlling the current that flows through a third terminal called the base. Understanding their operation requires delving into the fundamental principles of semiconductor physics and the behavior of minority and majority charge carriers.
There are two primary types of transistors: Bipolar Junction Transistors (BJTs) and Field-Effect Transistors (FETs).
1. Bipolar Junction Transistors (BJTs): BJTs consist of three layers of semiconductor material: the emitter, base, and collector. These layers are typically made of either N-type (excess electrons) or P-type (deficiency of electrons) semiconductor material. There are two types of BJTs: NPN (N-type emitter, P-type base, N-type collector) and PNP (P-type emitter, N-type base, P-type collector).
The working principle of a BJT involves the control of current flow between the emitter and the collector terminals by the injection of a small current at the base terminal. When a small current is applied to the base-emitter junction (forward bias), it allows current to flow across the base-collector junction. This phenomenon is governed by the majority and minority charge carriers in the semiconductor layers.
In an NPN transistor, for instance, electrons are the majority carriers in the N-type regions. When a positive voltage is applied to the base relative to the emitter, it creates a forward bias, allowing electrons to cross the base-emitter junction and enter the base. These electrons are minority carriers in the base region. A small number of these electrons recombine with the holes (deficient electrons) present in the P-type base region, leaving behind a larger number of holes. The remaining electrons diffuse and flow from the base to the collector, forming the collector current. This amplified collector current is a direct result of the small base current, making the BJT a current-amplifying device.
2. Field-Effect Transistors (FETs): FETs, on the other hand, operate by controlling the flow of current between the source and drain terminals using an electric field applied to a gate terminal. The key component here is the channel region between the source and drain, which can be thought of as a conductive pathway.
In Metal-Oxide-Semiconductor FETs (MOSFETs), the gate is insulated from the channel by a thin oxide layer. Applying a voltage to the gate terminal creates an electric field that influences the conductivity of the channel region. In an N-channel MOSFET, a positive voltage on the gate relative to the source attracts electrons towards the surface of the semiconductor material, creating a conductive channel for current flow between the source and drain terminals.
In P-channel MOSFETs, a negative voltage on the gate relative to the source attracts holes to the surface, allowing current to flow from source to drain. The voltage applied to the gate thus controls the conductivity of the channel, enabling FETs to act as voltage-controlled switches or amplifiers.
In summary, transistors operate by exploiting the behavior of semiconductor materials and the manipulation of charge carriers. Whether through the injection of minority carriers in BJTs or the modulation of channel conductivity in FETs, transistors form the bedrock of modern electronics, enabling the precise control and manipulation of electric current for a wide range of applications.
Bipolar Junction Transistors (BJTs)
Bipolar Junction Transistors (BJTs) are fundamental semiconductor devices that play a crucial role in electronic circuits, serving as both amplifiers and switches. Developed in the mid-20th century, BJTs revolutionized the field of electronics by providing a compact and efficient alternative to vacuum tubes. They are widely used in a plethora of applications, ranging from consumer electronics to industrial systems, owing to their ability to amplify weak signals and control large currents.
BJTs are constructed using three layers of semiconductor material: the emitter, base, and collector. These layers can be either N-type (excess electrons) or P-type (deficiency of electrons) silicon or other semiconductor materials. The arrangement of these layers determines the type of BJT, whether NPN or PNP.
NPN BJT:
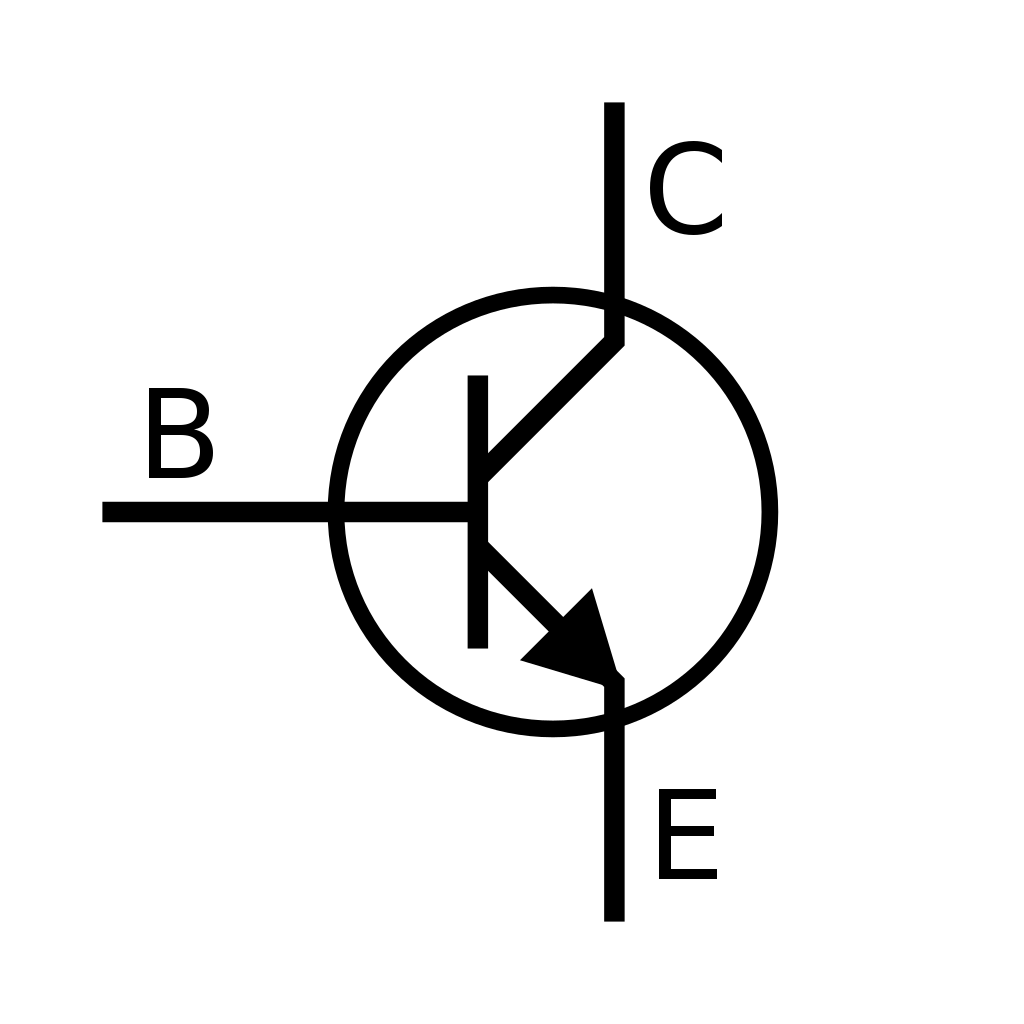
- Emitter: N-type semiconductor
- Base: P-type semiconductor
- Collector: N-type semiconductor
PNP BJT:
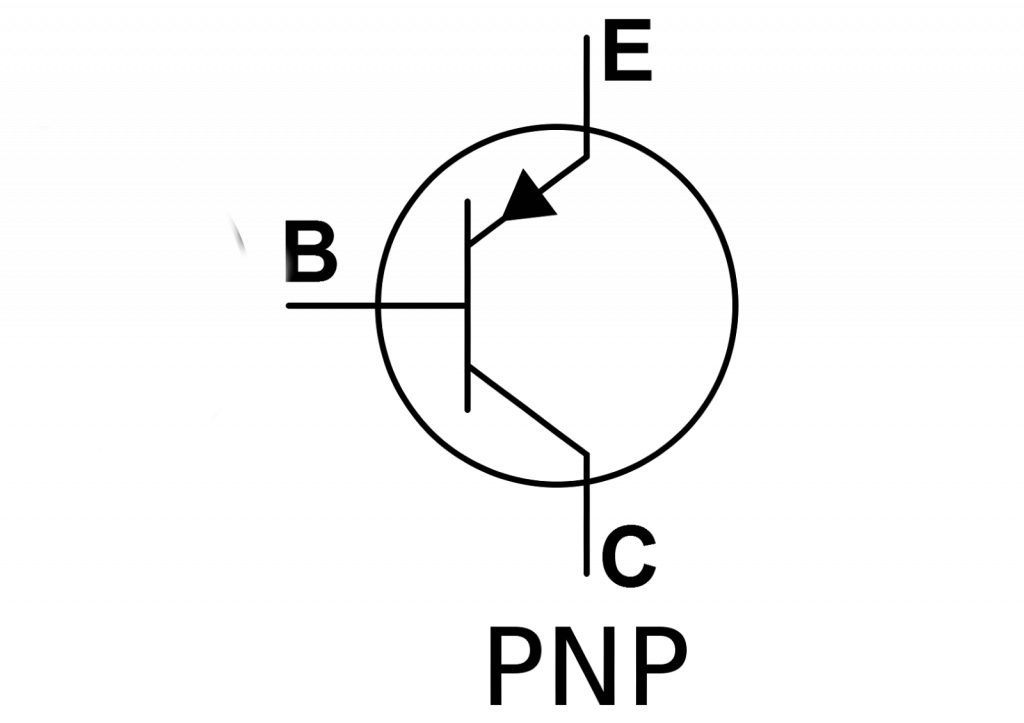
- Emitter: P-type semiconductor
- Base: N-type semiconductor
- Collector: P-type semiconductor
The operation of a BJT is based on the interaction of both majority and minority charge carriers in the semiconductor materials. There are two main modes of operation for a BJT: active mode (amplification) and cut-off mode (switching).
1. Active Mode (Amplification): In the active mode, the BJT operates as an amplifier. When a small current flows from the emitter to the base of an NPN transistor (or from the base to the emitter of a PNP transistor), it creates a flow of minority charge carriers (holes in the case of NPN and electrons in the case of PNP) in the base region. This forms a thin region where the number of charge carriers is much smaller than in the emitter or collector, known as the depletion region.
The minority carriers in the base diffuse towards the collector or emitter depending on the type of transistor. The majority carriers (electrons in NPN and holes in PNP) in the emitter and collector regions attract these minority carriers. This phenomenon results in the amplification of the small base current into a larger collector current. This process is often described using the term “transistor action,” where a small input current controls a larger output current.
2. Cut-Off Mode (Switching): In the cut-off mode, the BJT acts as a switch, allowing or preventing the flow of current between the collector and emitter. When there is no base current (or a very small base current), the transistor is in a cut-off state, and no current flows between the collector and emitter. This state is often compared to an open switch.
Controlling the base current allows for precise regulation of the collector current, making BJTs versatile components for signal amplification, current regulation, and logic functions in electronic circuits. However, it’s important to note that BJTs can be sensitive to temperature variations and have certain limitations in terms of power efficiency compared to other transistor types like Field-Effect Transistors (FETs).
Bipolar Junction Transistors are foundational elements in electronics, enabling the amplification and control of electrical signals. Their ability to amplify current and function as switches has made them integral to various applications across industries, shaping the way modern electronic devices operate and communicate.
PNP Transistors
PNP transistors are a type of bipolar junction transistor (BJT) that play a vital role in electronic circuits. They are designed with three layers of semiconductor material: the emitter, base, and collector. PNP transistors have applications in amplification, signal processing, and switching, much like their NPN transistor counterparts. However, their operation and characteristics are reversed due to the arrangement of their semiconductor layers.
In a PNP transistor:
- The emitter is made of P-type semiconductor material.
- The base is made of N-type semiconductor material.
- The collector is made of P-type semiconductor material.
The key difference between NPN and PNP transistors lies in the direction of the majority and minority charge carriers. In PNP transistors, the majority carriers are holes (absence of electrons), and the minority carriers are electrons. This reversal of charge carriers gives rise to distinct behaviors compared to NPN transistors.
1. Active Mode (Amplification): In the active mode, a small current flows from the base to the emitter of the PNP transistor. This forward-biases the base-emitter junction, allowing a flow of minority charge carriers (electrons) from the emitter into the base region. These electrons combine with the holes present in the P-type base, creating a thin region of low charge carrier concentration.
The majority carriers in the emitter and collector attract these minority carriers, resulting in the amplification of the small base current into a larger collector current. This amplification process allows PNP transistors to act as current amplifiers, signal processors, and voltage-controlled switches in electronic circuits.
2. Cut-Off Mode (Switching): In the cut-off mode, when there is no current flowing from the base to the emitter, the PNP transistor is in a non-conductive state. In this state, the majority carriers in the base block the flow of minority carriers, preventing current from passing between the collector and emitter. This is analogous to an open switch, where no current flows through the transistor.
PNP transistors find use in various applications, often in situations where NPN transistors might not be suitable. They can be used as high-side switches, which means they are placed in series with the load to control the positive supply voltage. This arrangement is particularly useful when dealing with positive ground systems or when a load needs to be disconnected from a positive voltage rail.
Overall, PNP transistors, along with NPN transistors, form a crucial part of electronic circuit design, enabling amplification, switching, and signal processing tasks. By understanding the behavior and characteristics of PNP transistors, engineers and designers can create sophisticated circuits that meet specific requirements across a wide range of applications.
Field-Effect Transistors (FETs)
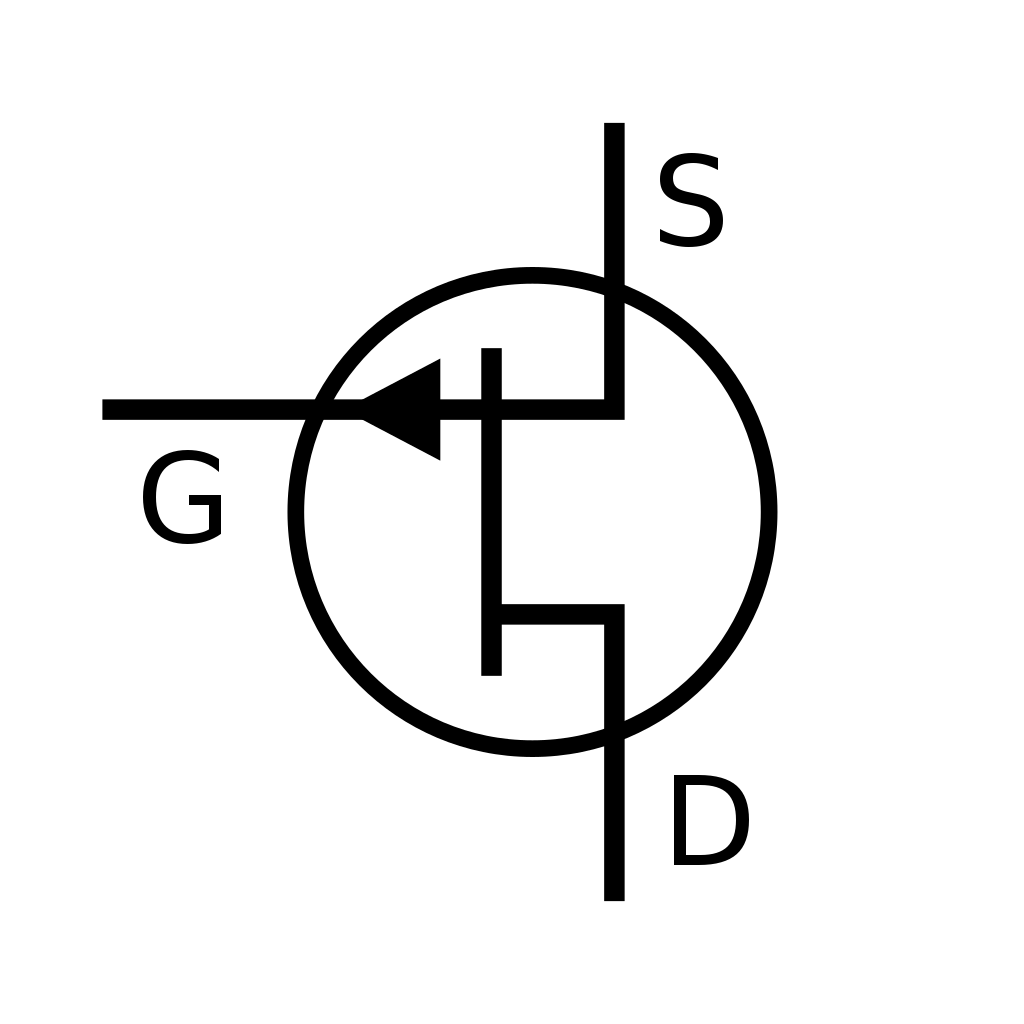
Field-Effect Transistors (FETs) are a category of semiconductor devices that are widely used for signal amplification, switching, and other electronic applications. FETs operate based on the control of an electric field within a semiconductor channel, which influences the flow of current between two terminals. Unlike Bipolar Junction Transistors (BJTs), FETs do not require the movement of charge carriers across junctions, making them more suitable for certain types of circuits and applications.
There are several types of FETs, but the two most common ones are Metal-Oxide-Semiconductor FETs (MOSFETs) and Junction Field-Effect Transistors (JFETs).
1. Metal-Oxide-Semiconductor FETs (MOSFETs): MOSFETs are perhaps the most prevalent type of FETs and have greatly influenced modern electronic devices and integrated circuits. They consist of a gate terminal, a source terminal, and a drain terminal. A thin insulating layer (usually an oxide layer, often made of silicon dioxide) separates the gate terminal from the channel region.
MOSFETs come in two main types based on the conductivity of the channel material: N-channel MOSFETs and P-channel MOSFETs.
- N-channel MOSFET: In this type, the channel is made of N-type semiconductor material. Applying a positive voltage to the gate terminal relative to the source creates an electric field that attracts electrons from the source to the channel, forming a conductive path between the source and the drain. This type of MOSFET is commonly used in electronic circuits due to its fast switching speeds and low on-resistance.
- P-channel MOSFET: Here, the channel is made of P-type semiconductor material. Applying a negative voltage to the gate terminal relative to the source forms an electric field that attracts holes to the surface of the channel, creating a conductive path. P-channel MOSFETs are often used in applications where they need to control the flow of positive voltage.
MOSFETs are known for their high input impedance, low power consumption, and their ability to provide excellent isolation between the gate and the channel. They are found in various electronic devices, including computers, mobile phones, power amplifiers, voltage regulators, and more.
2. Junction Field-Effect Transistors (JFETs): JFETs operate based on the control of the width of a depletion region in a semiconductor channel. They have three terminals: the gate, the source, and the drain. The gate-source junction is reverse-biased, creating a depletion region that widens or narrows based on the applied voltage.
JFETs come in two main types: N-channel JFETs and P-channel JFETs. By varying the reverse bias voltage applied to the gate, the width of the depletion region changes, affecting the current flow between the source and the drain. JFETs have higher input impedance compared to MOSFETs but are often slower in terms of switching speeds.
Field-Effect Transistors (FETs) are essential semiconductor devices used in a wide range of electronic applications. They offer advantages such as high input impedance, low power consumption, and efficient switching. Whether in the form of MOSFETs or JFETs, these devices have contributed significantly to the development of modern electronics and have enabled the creation of complex and energy-efficient electronic circuits.
Comparison between BJTs and FETs
Bipolar Junction Transistors (BJTs) and Field-Effect Transistors (FETs) are two distinct types of transistors used in electronic circuits for various purposes. Each type has its own set of characteristics, advantages, and limitations, making them suitable for different applications. Let’s compare BJTs and FETs based on several key factors:
1. Working Principle:
- BJTs: BJTs operate by controlling the flow of both majority and minority charge carriers (electrons and holes) through a semiconductor material. They are current-controlled devices.
- FETs: FETs operate by controlling the flow of current through a semiconductor channel using an electric field. They are voltage-controlled devices.
2. Voltage vs. Current Control:
- BJTs: BJTs are primarily controlled by the current flowing into their base terminal. Changes in base current lead to amplified changes in collector current.
- FETs: FETs are primarily controlled by the voltage applied to their gate terminal. Changes in gate voltage influence the current flowing through the channel between the source and drain terminals.
3. Input Impedance:
- BJTs: BJTs have lower input impedance compared to FETs. This means that they draw more input current and can load down input signals.
- FETs: FETs have much higher input impedance, making them less sensitive to loading effects and better suited for high-impedance applications.
4. Amplification:
- BJTs: BJTs provide higher current gain (current amplification) compared to FETs. They are commonly used in analog amplification circuits.
- FETs: FETs have voltage amplification characteristics and are often used in high-frequency applications due to their low noise and high input impedance.
5. Speed and Switching:
- BJTs: BJTs generally offer faster switching speeds than FETs, making them suitable for high-speed applications.
- FETs: FETs have slower switching speeds compared to BJTs, but advancements have been made in high-frequency FETs that perform well in RF applications.
6. Power Efficiency:
- BJTs: BJTs are less power-efficient compared to FETs, as they dissipate more power in their on-state due to the voltage drop across the collector-emitter junction.
- FETs: FETs are more power-efficient as they have minimal voltage drop across the channel, resulting in lower power dissipation in the on-state.
7. Noise Performance:
- BJTs: BJTs tend to have higher noise levels due to the presence of thermal noise generated by the movement of charge carriers.
- FETs: FETs exhibit lower noise levels, making them preferable in applications where noise performance is critical, such as in low-noise amplifiers.
BJTs and FETs have distinct characteristics that make them suitable for different types of electronic circuits and applications. BJTs are often chosen for their current amplification and fast switching capabilities, while FETs excel in high-impedance and low-noise applications. Engineers select the appropriate transistor type based on the specific requirements of their circuits, balancing factors like speed, power efficiency, impedance, and noise performance.
Applications of Transistors
Transistors are versatile semiconductor devices that have transformed the landscape of modern electronics and enabled an extensive array of applications. Their ability to amplify signals, control current flow, and act as switches has revolutionized various industries. Here are some key applications of transistors:
1. Amplification: Transistors are extensively used in amplification circuits to boost weak electrical signals, making them suitable for communication systems, audio equipment, and medical devices. By connecting multiple transistors in various configurations, engineers can achieve precise and controlled signal amplification.
2. Signal Processing: Transistors play a central role in signal processing circuits such as filters, oscillators, and modulators. These circuits are vital for tasks like frequency tuning, noise reduction, and signal modulation in radio, television, telecommunications, and music equipment.
3. Switching: Transistors are widely used as switches in digital circuits. By controlling the base current (for BJTs) or the gate voltage (for FETs), transistors can be turned on or off, allowing them to act as binary switches in digital logic gates, microcontrollers, and integrated circuits.
4. Voltage Regulation: Transistors are used in voltage regulator circuits to stabilize and regulate output voltages. They are crucial components in power supplies, battery charging systems, and voltage control modules.
5. Oscillators and Clock Generators: Transistors are essential for generating stable oscillations in electronic circuits. They are used in oscillator circuits that produce periodic waveforms for applications like generating clock signals in digital systems and producing radio frequency signals.
6. Audio Amplification: Transistors are used in audio amplifiers to amplify audio signals for loudspeakers and headphones. They help enhance the quality and volume of audio output in devices such as radios, music players, and home theater systems.
7. Digital Logic Circuits: Transistors are the building blocks of digital logic circuits. They enable the creation of complex systems for digital computing, memory storage, and processing tasks in computers, smartphones, and other digital devices.
8. Radio Frequency (RF) Communication: Transistors are vital in RF amplifiers and mixers for wireless communication devices such as cell phones, Wi-Fi routers, satellite communication systems, and radar equipment.
9. Power Control and Conversion: Transistors are employed in power control circuits like motor drivers, power inverters, and voltage converters. These circuits find applications in robotics, automation, electric vehicles, and renewable energy systems.
10. Sensing and Measurement: Transistors are used in sensor circuits to detect changes in environmental factors such as temperature, light, pressure, and proximity. These sensors are used in applications like weather monitoring, industrial automation, and consumer electronics.
11. Medical Devices: Transistors are found in various medical devices, including pacemakers, EEG machines, and ultrasound equipment. They contribute to signal amplification, signal processing, and control in these critical applications.
12. Aerospace and Defense: Transistors are integral to avionics, communication systems, radar systems, and missile guidance systems used in aerospace and defense applications.
In essence, transistors have transformed virtually every aspect of modern life by enabling innovations in technology, communication, automation, and more. Their versatile functionality continues to drive advancements in electronics and shape the evolution of various industries.
Conclusion
Transistors stand as the fundamental pillars of modern electronics, ushering in an era of unparalleled technological advancement. These ingenious semiconductor devices, through their ability to amplify, switch, and control electric currents, have underpinned the development of countless innovations that shape our daily lives. From the basic yet powerful Bipolar Junction Transistors (BJTs) to the voltage-controlled Field-Effect Transistors (FETs) with their diverse configurations, the transistor family has brought forth a multitude of capabilities, each tailored to meet the specific demands of various applications.
The journey through the world of transistors has unveiled the intricate interplay between charge carriers, semiconductor materials, and electrical fields that form the basis of their operation. This understanding has led to the creation of amplifiers that capture delicate signals, switches that seamlessly control electronic pathways, and intricate integrated circuits that drive the digital age. The versatility of transistors is evident in their diverse applications – from the communication systems connecting people across the globe, to the medical devices saving lives, and the cutting-edge technologies propelling space exploration.
As technology continues to evolve, so too will the realm of transistors. Advancements in material science, miniaturization techniques, and innovative circuit designs will further push the boundaries of what is possible. The ongoing quest for faster, more energy-efficient transistors will continue to shape the trajectory of electronics and drive us toward a future where the unimaginable becomes reality.
In essence, the world of transistors and their types encapsulates the essence of human ingenuity – a fusion of science and engineering that has revolutionized our world in profound ways. From the early days of their discovery to the complex integrated circuits of today, transistors remain at the heart of our technological endeavors, promising a future brimming with possibilities yet to be realized.