Electricity is a fundamental aspect of the natural world that has shaped the way we live and interact with technology. At its core, electricity is the result of the movement of charged particles, known as electrical charges. These charges can be either positive or negative and are responsible for the creation of electric currents, which power everything from our homes to the most advanced electronic devices.
The concept of electrical charges dates back to ancient times when the Greeks discovered that rubbing certain materials together, such as amber and fur, could produce an attractive force between them. This phenomenon demonstrated the presence of two types of charges, which were later named positive and negative.
Understanding electrical charges and the flow of electric currents is crucial in various scientific, technological, and practical applications. From the illumination of light bulbs to the operation of complex computer systems, electrical currents play a pivotal role in modern society.
In this exploration of electrical charges and current, we will delve into the foundational principles of electricity. We will discuss the nature of charges, how they interact with one another, and how these interactions give rise to electric currents. Additionally, we will touch upon the historical milestones that have led to our current understanding of electricity, as well as the essential mathematical and physical concepts that underpin the field.
Whether you’re a curious beginner seeking to grasp the basics of electricity or someone looking to refresh their knowledge, this journey into electrical charges and current will provide you with the insights you need to comprehend the intricate world of electrical phenomena and its significance in our lives. So, let’s embark on this enlightening exploration of electrical charges and current to unveil the mysteries and marvels of the electrifying realm that surrounds us.
Fundamentals of Electrical Charges
Electric charges are the foundation of electricity and are responsible for the interactions and phenomena that we associate with the flow of electricity. They are intrinsic properties of matter and come in two types: positive and negative. The behavior of these charges and their interactions with each other form the basis of understanding electrical phenomena.
1. The Nature of Electric Charges: Electric charges are properties of subatomic particles, such as protons, electrons, and ions. Protons carry a positive charge, while electrons carry a negative charge. The charge of an electron is equal in magnitude but opposite in sign to that of a proton. This duality is essential for maintaining the neutrality of atoms, where the number of protons (positive charges) in the nucleus is balanced by the number of electrons (negative charges) in orbit around it.
2. Conservation of Electric Charge: One of the fundamental principles of electric charges is the conservation of charge. This means that electric charge cannot be created or destroyed; it can only be transferred from one object to another. When an object gains electrons, it becomes negatively charged, and when it loses electrons, it becomes positively charged. The total amount of charge in a closed system remains constant.
3. Like Charges Repel, Opposite Charges Attract: According to Coulomb’s law, two objects with the same type of charge (both positive or both negative) will repel each other, while objects with opposite charges will attract each other. This principle is at the heart of the interactions between charged particles.
4. Quantization of Charge: Charge is quantized, meaning it exists in discrete units. The smallest unit of charge is the elementary charge, which is the charge carried by a single electron or proton. All other charges are integer multiples of the elementary charge.
5. Conductors and Insulators: Materials can be classified as conductors or insulators based on their ability to allow the movement of charges. Conductors, such as metals, have electrons that are relatively free to move throughout the material, facilitating the flow of electric current. Insulators, on the other hand, have tightly bound electrons and do not allow easy charge movement.
6. Charging Mechanisms: There are several ways to charge objects, including friction (rubbing), conduction (direct contact), and induction (influencing without direct contact). These mechanisms involve the transfer of electrons between objects, leading to a redistribution of charges.
Understanding these fundamental aspects of electric charges is crucial for comprehending the behavior of electricity in various systems and applications. From the simple concept of attracting and repelling charges to the complex interactions within electronic devices, the knowledge of electric charges forms the cornerstone of our understanding of electrical phenomena.
Nature of Current Electricity
Current electricity is a dynamic and essential aspect of modern life, powering a vast array of devices and systems that have become integral to our daily routines. It is the movement of electric charges through a conductor, such as a wire, that gives rise to electric current. Understanding the nature of current electricity is key to grasping its practical applications and the principles that govern its behavior.
1. Electric Current and Charge Flow: Electric current refers to the flow of electric charges within a material. In most cases, this involves the movement of electrons, which are negatively charged particles. Current flows from areas of high potential (positive charge) to areas of low potential (negative charge). However, due to historical conventions, current is considered to flow in the opposite direction, from positive to negative.
2. Current Measurement: The unit of electric current is the ampere (A), often simply referred to as “amp.” One ampere of current corresponds to the flow of one coulomb of charge per second. This relationship underscores the concept of current as a rate of charge flow.
3. Direct and Alternating Current: Electric currents can be categorized into two main types: direct current (DC) and alternating current (AC). In DC, the flow of electric charges remains constant in one direction, as seen in batteries and most electronic devices. AC, on the other hand, involves the periodic reversal of charge flow direction, typically occurring in power distribution systems and household circuits.
4. Factors Affecting Current: Several factors influence the magnitude of electric current in a circuit:
- Voltage (Potential Difference): Voltage is the driving force that causes charges to flow. A higher voltage across a circuit results in a greater current.
- Resistance: Resistance is a measure of how much a material resists the flow of current. It depends on the material’s properties and dimensions. According to Ohm’s law (I = V/R), current is inversely proportional to resistance for a given voltage.
- Temperature: The resistance of most conductors increases with temperature. This phenomenon can impact current flow in circuits.
5. Ohm’s Law and Electrical Circuits: Ohm’s law, named after the German physicist Georg Simon Ohm, is a fundamental principle in the study of electric circuits. It states that the current passing through a conductor is directly proportional to the voltage applied across it and inversely proportional to the resistance of the conductor (I = V/R).
6. Series and Parallel Circuits: Components in electrical circuits can be connected in series or parallel configurations. In a series circuit, the same current flows through all components, while in a parallel circuit, the voltage across all components is the same, but the current can vary.
7. Electrical Power: The rate at which electrical energy is converted or delivered is measured in watts (W). Power (P) can be calculated using the formula P = IV, where I is the current and V is the voltage across a component.
The nature of current electricity extends from the basic principles of charge movement to the intricacies of circuit behavior and power distribution. Whether it’s powering our homes, lighting up our cities, or fueling our electronic devices, current electricity is an indispensable part of modern society, underpinned by the deep understanding of the flow of electric charges.
Electric Fields and Potential Difference
Electric fields and potential difference are fundamental concepts in the study of electricity that help us understand the behavior of charged particles and the interactions between them. These concepts are crucial for comprehending how electric charges influence their surroundings and how energy is transferred in electrical systems.
1. Electric Fields: An electric field is a region of space around a charged object where another charged object would experience a force. Electric fields are created by electric charges and are characterized by their direction and strength. The strength of an electric field at a point is defined as the force experienced by a unit positive charge placed at that point.
- Direction of Electric Field: Electric field lines point away from positive charges and toward negative charges. The density of field lines indicates the strength of the field, with denser lines indicating a stronger field.
- Mathematical Representation: Electric fields are represented using vectors. The electric field (E) at a point is the force (F) experienced by a test charge (q) placed at that point, divided by the magnitude of the test charge: E = F/q.
2. Potential Difference (Voltage): Potential difference, often referred to as voltage, is a measure of the electric potential energy difference between two points in an electric field. It is the work done per unit charge to move a charge from one point to another against the electric field.
- Voltage and Energy: Charges move in response to voltage differences. If a charge is moved within an electric field in the direction of increasing voltage, it gains potential energy. Conversely, if it moves in the direction of decreasing voltage, it loses potential energy.
- Unit of Voltage: The unit of voltage is the volt (V). One volt is defined as one joule of energy per coulomb of charge.
3. Relation Between Electric Field and Voltage: The relationship between electric field (E) and voltage (V) is given by the equation: V = Ed, where d is the distance between the two points with the potential difference. This equation reflects the fact that electric field strength is a measure of how quickly voltage changes over a given distance.
4. Equipotential Surfaces: An equipotential surface is a surface on which the electric potential is the same at every point. No work is required to move a charge along an equipotential surface because the potential difference is zero. Electric field lines are always perpendicular to equipotential surfaces.
5. Capacitors and Potential Difference: Capacitors are devices that store electrical energy in the form of potential difference between their plates. When a potential difference is applied across the plates of a capacitor, positive and negative charges accumulate on the respective plates, creating an electric field between them.
Electric fields and potential difference are fundamental concepts in the study of electricity and play a critical role in various applications, including the design of electrical circuits, electronic devices, and power distribution systems. These concepts allow us to understand how charges interact and how energy is harnessed and transferred within electrical systems.
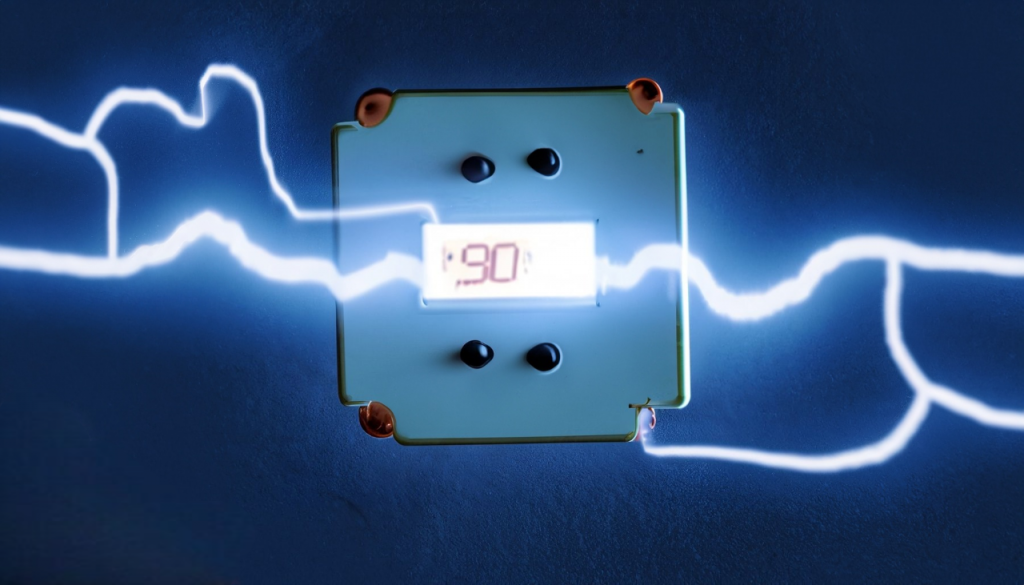
Conductors and Insulators
Conductors and insulators are two distinct classes of materials that exhibit contrasting behavior when it comes to the flow of electric charges. These materials play a pivotal role in the field of electricity, influencing how currents propagate and circuits operate. Understanding the differences between conductors and insulators is essential for designing and optimizing electrical systems.
Conductors:
Conductors are materials that allow electric charges to move freely through them. This property arises from the presence of mobile charged particles, typically electrons, that can easily flow in response to an applied electric field. In conductors, the outermost electrons of atoms are loosely bound to their nuclei, enabling them to move between atoms and carry electric current.
Key characteristics of conductors include:
- High Electrical Conductivity: Conductors have a high conductivity, which is a measure of how easily electric charges can move through the material. Metals, such as copper, silver, and aluminum, are excellent conductors due to their atomic structure and the mobility of their electrons.
- Low Resistance: The resistance of a conductor to the flow of electric current is relatively low. This low resistance allows for efficient current flow and minimal energy loss as heat.
- Electrostatic Equilibrium: The charges within a conductor will quickly redistribute themselves to reach electrostatic equilibrium, where the electric field inside the conductor is zero. This property ensures that charges are distributed uniformly on the surface of the conductor.
Insulators:
Insulators, also known as dielectrics, are materials that inhibit the movement of electric charges. In insulators, electrons are tightly bound to their atoms, making them less mobile compared to those in conductors. As a result, insulators do not readily conduct electric current.
Key characteristics of insulators include:
- Low Electrical Conductivity: Insulators have very low electrical conductivity. They resist the flow of electric charges and do not allow current to pass through easily.
- High Resistance: The resistance of insulators is high, which means that they do not facilitate efficient current flow. This property makes them suitable for applications where isolation and prevention of current leakage are essential.
- Dielectric Properties: Insulators are commonly used as dielectrics in capacitors and other electrical devices. When subjected to an electric field, the atoms in insulators become polarized, leading to the storage of electrical energy.
Examples of insulators include rubber, glass, plastic, wood, and ceramics. These materials find use in various applications, such as electrical insulation, protection from electric shocks, and the creation of barriers between conductive materials.
The distinction between conductors and insulators has far-reaching implications in electrical engineering and everyday life. Whether it’s the wiring in our homes, the insulation of power lines, or the operation of electronic devices, the careful selection and understanding of these materials contribute to the safe and efficient utilization of electrical energy.
Conclusion
In the world of electricity, the concepts of electrical charges and current serve as the foundation upon which our understanding of the natural and technological realms is built. Through our exploration of these fundamental concepts, we’ve uncovered the intricate dance of electrons, the interactions of charges, and the pathways through which energy flows to power our lives.
From the ancient observations of static electricity to the advanced technologies of today, our comprehension of electrical charges has evolved significantly. We’ve come to appreciate the nature of positive and negative charges, their behaviors, and the laws that govern their interactions. Electric charges, both static and in motion, have shaped our modern world, enabling the communication, transportation, and conveniences we often take for granted.
Electric current, the dynamic movement of these charges, has revolutionized society by illuminating our homes, connecting us through global networks, and propelling innovation in countless fields. The principles of current flow, from the foundational understanding of electric fields and potential difference to the intricate nuances of circuits and components, have empowered engineers, scientists, and inventors to shape the world as we know it.
As we conclude this exploration of understanding electrical charges and current, we recognize the vital role these concepts play in our daily lives and the broader scope of scientific inquiry. Whether it’s the hum of an electronic device or the humbling beauty of a lightning storm, the dance of charges and the flow of current continue to captivate our imagination and drive the progress of human knowledge and achievement. Embracing the wonders of electricity enriches our understanding of the universe and empowers us to create a future where the potential of electric charges and currents is harnessed for the betterment of humanity.
Recent Comments